The Uranium Story
Welcome, subscriber. I'd like to start by telling you a story. A story about energy. As with all forms of energy, this story begins with a star.
Once upon a time, a massive star reached the end of its life. Hydrogen ran low. Helium fused to beryllium. The final reaction: silicon fused to iron. The fusion reaction that powered its brilliant core slowed, dimming red as iron formed in the core sapped the reaction of crucial energy. After eons of elegant balance, the forces of gravity won out over the pressure of radiation. Flares dotted the surface, signaling the collapse of the outer magnetic field as a critical threshold was finally reached. In a single dramatic moment, the star collapsed under its weight.
As the star's core collapsed, the nuclei of heavy fusion products raced to the center, accelerating ever faster, until forces we can barely comprehend violently smashed nucleus into nucleus, compressing the core into a tiny raging particle storm.
Neutrons became protons. Neutrinos flew out in every direction. Strong nuclear forces pulled the particle soup into larger, heavier nuclei that could never form under normal fusion. Finally, the soup reached a critical pressure and the whole mess exploded outward in a violent supernova and hurled fragments of core outwards at incredible speeds.
As these fragments of core flew out, the particle storms within coalesce into heavy atoms, binding the energy of the dying star into solid form. Many of those fragments ended up in the cloud of preplanetary material that would one day become Earth and the other planetary bodies of our solar system. As the planet cooled, these heavy elements formed into rich veins of ore to one day be dug up to build the foundations of human civilization. Copper. Nickel. Silver. Gold. Platinum.
Uranium.
Some say it's the key to the survival of humanity. Others say it'll be the doom of us all. Many simply don't know enough to form an opinion. No matter where you stand, one thing is clear: uranium is here to stay (at least for the next 3.5 billion years), and it's time you knew more about it. As this is my first free article, it will provide a high-level educational overview of uranium as an element and an industry.
Natural Uranium
To understand why uranium is important, let’s talk a little science. First things first: let’s clear up a little unfortunate misconception brought on by shows like The Simpsons. Uranium does not naturally glow green. At least, not under its own energy. If it did, it’d be much easier to find. It will glow under high-frequency light, but otherwise most naturally-occurring uranium ore just looks like granite.
Some forms, such as tobernite or carnotite may occur as dramatic green or yellow crystals that are easily identified, but the majority are black, brown, gray, or red stones, indistinguishable from granite to the untrained eye without the aid of instruments like a Geiger counter.
Locked within this unassuming rock is natural uranium ore. Due to the radioactive nature of uranium, natural is a very specific term not used to describe most other metals. (When’s the last time you heard someone refer to “natural” iron?). For uranium, the term “natural” refers to the balance of protons and neutrons within the nucleus of every atom of the metal. By definition, all uranium atoms have 92 protons, the positively charged fundamental nuclear particles. If this number was different, it would not be uranium.
The crucial property separating the different “types” (isotopes) of uranium we care about is the number of uncharged neutrons in the nucleus. 99.27% of natural[1] uranium atoms contain 146 neutrons, and are known as Uranium-238, U-238, or 238U. A tiny fraction, less than 0.01%, contain 142 neutrons, forming U-234. The remaining 0.72% contains 143 neutrons, forming Uranium-235, the isotope we care about. Unlike U-238 and U-234, U-235 has a high probability of fission, whether by spontaneous decay or in the aftermath of absorbing an incoming neutron.
A key concept to understand here: in the fission of heavy elements like uranium, the original whole is greater than the sum of the fragments. How? While it’s easy to think of nuclei as invariant sums of the mass of their protons and neutrons, this isn’t quite true. The strong nuclear force holding the neutrons and protons together in atomic nuclei results in a binding energy which has “its own mass,” so when all the protons and neutrons are together, the total binding energy of all those things packed close together results in a “heavier” nucleus.
When it breaks, that energy has to go somewhere. (Think E=mc2). As that energy is released, it’s converted to kinetic energy (which becomes heat), photons (like gamma or x-rays), or neutrinos. On average, breaking a single nucleus of U-235 yields 200 MeV (megaelectron-volts) of energy. If you’re not a physicist, I’m certain that number means nothing to you. That’s fine. To put it in more everyday terms, if you broke every nucleus in one kilogram of U-235, it would yield 82 terajoules – the energy equivalent of 2.5 million liters of gasoline. Such incredible energy density presents the incentive for finding, refining, and enriching uranium into nuclear fuel.
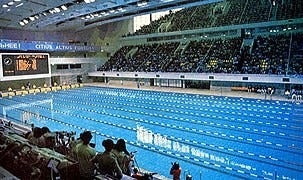
Mining for Uranium
I mentioned uranium is hard to find. The methods for doing so affect the chances of success for those who prospect for it, but those are a little beyond the scope of this article. Simple version though: they use Geiger counters. A lot of them. Once a prospective site is found, though, the march of mine development begins, starting with junior miners.
A Junior Miner is the modern term for a prospector who claims land rights and explores the land for the desired resource. Once they find it, they typically sell the rights to Intermediate Miners, who develop the site of the discovery and ready it for production. Then they sell it to Major Miners who begin and execute large-scale production from the site.
The whole process is basically flipping properties until the end, but with mines instead of houses. I’ll go into more detail in the next article. For now, the basics:
Junior miners are a dime a dozen, and run the range from a guy with just a shovel and a Geiger counter, to sophisticated teams with specialized drilling and sensing equipment. Usually you can only find them trading on the Australian stock exchanges. Some may be sitting on absolute gold mines of 7% ore. Most just get the little sniffs of radon seeping from 0.2%ish ore. Some push the limits of fraud under a variety of covers. Many end up going bankrupt. That’s the price to play. The ones who win though – they do one of two things:
They hire an additional geologist or two and a few experts to help create a compelling pitch to sell the mine to an intermediate or even major miner
They hire a bunch more geologists and experts to help create a compelling pitch to attract investments as a new intermediate miner on the scene
Either way, junior mining is pay-to-play. Once they’ve “proven” the uranium is in the ground and handed the mine off to the next stage, mine development begins. Uranium mining today is a little different than some other ore mining, though.
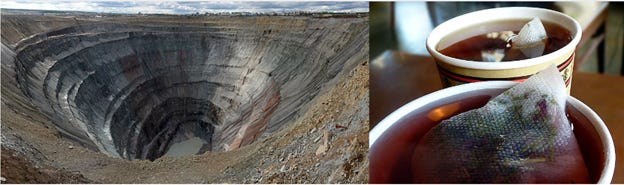
During the early years of uranium mining, it was excavated in hard rock form in traditional open pits, then later in underground tunnels[2]. Oftentimes, uranium was just a byproduct in the quest for other metals like silver, vanadium, radium, or copper. Today, those methods are largely defunct, and most uranium mining occurs via the In-Situ Leach process, or ISL. Instead of opening a giant hole in the ground, pipes are driven into suitable locations, then a solvent is injected into the ground, slowly circulated through to dissolve uranium out of the ore, and then extracted and stored as a uranium-bearing solution. This provides one huge advantage.
ISL provides a large reduction in solid mine tailings compared to physical rock mining by combining most of the milling steps into the mining process. Milling is normally the process by which solid mined ore gets crushed into powder, sorted, dissolved in acid or another suitable solvent, then treated to get the desirable product out of the resulting solution, and most mills are operated by major miners. What’s left over – nonmetallic ore rock, sludge from the precipitated solution, random debris – is rejected to a powdery pile known as the tailings. In the case of uranium, the mine tailings are usually radioactive, so it’s rather undesirable to store heaps of it on the surface.
ISL mining mostly removes this concern by leaving the tailings in the ground, since the mining process is actually most of the chemical milling process. With open pit mines, your output is dependent on the operation of a separate crushing and processing mill. With ISL mining, your output is just a function of chemistry, and goes through much less heavy hardware than a traditional crushing process.
Once the concentrated uranium solution is obtained, it’s further reacted, separated, and baked until it eventually turns into U3O8, a uranium oxide known as yellowcake. This form is what’s traded on the open market when you hear about pounds of uranium being traded as a commodity. It looks like this:
Once it’s in this form, it gets traded to many different destinations. Most of it gets sold to fuel producers. Some gets packed into barrels and shipped off to the vaults of the Sprott Physical Uranium Trust, never to be seen on the market again. In either case, when you see the price of uranium being quoted as $X/lb, this price is referencing the cost per pound of U3O8 being moved about, not pure uranium metal. The price of uranium doesn’t just end at pounds of yellowcake, though. In order to be useful to humanity, the yellowcake has to be converted and then enriched[3].
Enrichment (and other factors in the price of U)
Remember how we said natural uranium only contains 0.72% U-235? This is called the enrichment level. The majority of uranium end customers are utility-scale nuclear reactor operators. Most of their reactors are cooled by light water coolant, and they have two problems with natural yellowcake: first, yellowcake is not suitable for use as nuclear reactor fuel, and second, natural uranium has too low of an enrichment to be used as fuel in most reactors to begin with, since light water reactors typically require a minimum enrichment of 3% or higher to function properly. Specialized research reactors may demand fuel enrichments of up to 20%. This is where economics beyond the trade price of yellowcake begin to define the real cost of uranium.
Recall that the difference between U-235 and U-238 is the presence of three more neutrons in the nucleus. This makes a single atom of U-238 ever so slightly heavier than an atom of U-235. Chemically, there is no way to separate them, since isotopes of any element are chemically identical to all other isotopes of the element, so fuel fabrication requires some other way to turn the natural concentration of uranium to concentrated fuel. The easiest, most cost-effective way today is by gas centrifuge.
To begin this process, the yellowcake undergoes conversion. The yellowcake is reacted with fluorine gas to form a new solid: UF6. Unlike yellowcake though, when UF6 is heated, it changes from solid to gas. In this form, it can get spun in long chains of centrifuges at high speeds to sort the lighter U-235 from the common U-238, taking advantage of the barely different densities of the two gases to skim U-235 from the middle and pass it on to the next centrifuge in the chain, on and on until the desired enrichment level is reached.
All those centrifuges require power to operate, and sometimes the enrichment facility may not have enough work to keep those centrifuges spinning. The cost of shutdowns and startups of these large plants can be prohibitively expensive, so to prevent idling a bank of centrifuges, enrichers will use strategies known as underfeeding or overfeeding.
In general, underfeeding is much more common, and it causes a downward pressure on the price of uranium since it results in oversupply of enriched uranium if downstream demand does not change over time. This effect is much less pronounced in the gas centrifuge process since it costs much less to operate than other methods, but the effect is still there. The cost to operate the enrichment plants is expressed in cost per Separative Work Unit, or SWUs.
The SWU is a complex unit dependent on several factors, and will be covered in a later article. For now, know that it’s the standard unit of billing for enrichment services, and it’s another factor in the final price of uranium fuel. When the uranium gas reaches the target enrichment, it is cooled back down into a solid to be turned into fuel.
End Use
At the final end of the uranium industry is the nuclear fuel sector. The final enriched product from the centrifuges is further reacted with chemicals to obtain a form of uranium suitable for use as a nuclear fuel. Some reactors are capable of using uranium metal, but most only use uranium oxide ceramics as fuel, so UF6 is converted to UO2. Those ceramics are pressed into pellets. The pellets are arranged into hollow rods to form fuel rods. Fuel rods are combined into fuel assemblies, and fuel assemblies are combined in a metal bowl to create a nuclear reactor.
Under the right, carefully-controlled conditions, a nuclear reactor will “burn” the uranium to produce heat and neutron flux in a slow, long-running cycle. The heat will be captured and transported to be used mainly as process heat, or converted to electricity. Certain reactors will also make use of the neutron flux to produce short-lived radioactive isotopes critical to the functioning of our modern world – x-ray sources for medicine, radioactive tracers in injected dyes, radiographic sources for piping inspections, and so on.
Sounds like a much better way to get energy than setting a pool of gasoline on fire, no? And to think – it all came from a star that collapsed long ago.
The Takeaway
If nothing else, my goal is for you to come away from this with at least a basic understanding of the industry, summarized into a few sentences or less.
How the Uranium Industry Makes Money: Solvent is pumped into the ground to dissolve uranium and bring it to the surface. It is then treated with chemicals to form yellowcake, treated with more chemicals to form a gas, then centrifuged to obtain higher concentrations of the lighter U-235 for use as fuel. The enriched gas is condensed to form nuclear fuel, and that fuel is burned in nuclear reactors to produce heat and specialized isotopes.
This concludes my high-level overview of uranium. If you’re interested in the upcoming deep dives into the individual sectors of the uranium industry, subscribe and let us know which part you’d like to hear about first!
[1] Conventionally, this number excludes a large deposit of depleted uranium in Gabon known as the Oklo Natural Reactor.
[2] This was before they knew this was a bad idea due to how it gave you cancer from chronic radon exposure.
[3] Unless you’re fueling a CANDU. Then you don’t enrich. You just make natural uranium fuel.
Readers, let me know which sector you find most interesting for the closer look in the next article. What would you want to understand better?